All published articles of this journal are available on ScienceDirect.
The Use of LASER and its Further Development in Varying Aspects of Surgery
Abstract
Lasers are now common place in day to day life and have been used across the sciences since their description as far back as 1917. Initially met with some sceptism, their use in medicine and in particular the surgical specialty has only increased. Their use in the future is also likely to expand as we continue to improve their efficiency and technology surrounding them.
This review aims to introduce lasers to the novice. The topic of lasers is vast and complex, but we aim to give enough information for the reader to understand how lasers are designed, how they were created, as well as a summary of their current use in medicine.
INTRODUCTION
The acronym laser or LASER stands for “light amplification by stimulated emission of radiation” and its name accredited to Gordon Gould in 1957. Before this time the laser represented the ‘optical MASER,’ another acronym meaning “microwave amplification by stimulated emission of radiation.” However, both applications found their origins at the beginning of that century and in 1917 most famously, Albert Einstein put forward his theory of ‘stimulated emission’.
This review aims to introduce lasers to the novice. The topic of lasers is vast and complex, but we aim to give enough information for the reader to understand how lasers are designed, how they were created, as well as a summary of their current use in medicine.
LASER; THE PHYSICS
Visible light describes the form of electromagnetic radiation whose waves are visible to the human eye. Out with this range, the electromagnetic spectrum continues and amongst others include ultraviolet and infrared wavelength forms. Electromagnetic radiation is absorbed as it travels, particularly by biological tissues, and when absorbed can be described as having photochemical or thermal properties. Excessive radiation energy can also destroy tissue resulting in a mechanical effect.
Light will travel in a straight line, or ray, unless it is disturbed. In a general space, this light disperses and so reduces its intensity. The idea behind lasers is maximising the concentration of light for an end ‘destructive force.’ This is created by the use of a single colour, or wavelength, through a narrow beam and allowing an additive effect on its destination.
These concepts are fundamental in laser use and are described as creating a ‘monochromatic,’ ‘collimated’ and ‘coherent’ light.
By using light of a specific wavelength, absorption is minimised except for the targeted substance. Specifically a laser light is coherent (all the light waves are in phase) and monochromatic (single wavelength). Furthermore, they travel in the same direction and are therefore said to be collimated. Irradiance is another term referring to the high radiant potential of the laser per unit area, due to its concentration in a narrow special band.
The laser light is created by stimulating a known substance (media) to absorb energy. As this substance is excited, the electrons in the media move to a higher energy level. This unstable state causes the electron to return to its normal state immediately and in doing so it emits energy in the form of a photon. The term ‘population inversion’ describes the number of atoms in the media in the excited state versus the number in their normal, or ground, state.
If this photon stimulates a similar media atom still in its unstable state a further photon is released, and so stimulated emission of photons is created. As the media is made of specific atoms, the photons released by each atom are of a single wavelength. This ‘knock on’ effect allows the subsequent same wavelength photon to be released in the same direction and at a certain time allowing coherence through the whole media.
LASER; LASING
The media of a laser is excited by firing a light (pumping) through it. The photons generated by the media, are then reflected within a ‘cavity’ of mirrors resulting in an amplification of the photons, and thus energy. This cascade effect moves the photons down the laser until they reach a partial mirror where some of the photons are released. This forms the laser output, and when focused can result in a higher power density. The light produced in this process is concentrated in a very narrow spectrum of wavelengths and the purest monochromatic light available (Fig. 1).
The medium, or ‘lasing media,’ is required to be excited to release photons. This medium is usually either a gas, liquid, crystal or a semiconductor. There are various methods of exciting the lasing medium including high voltage discharge, continuous light, light from a flash lamp, radio-frequency radiation and even light from another laser.
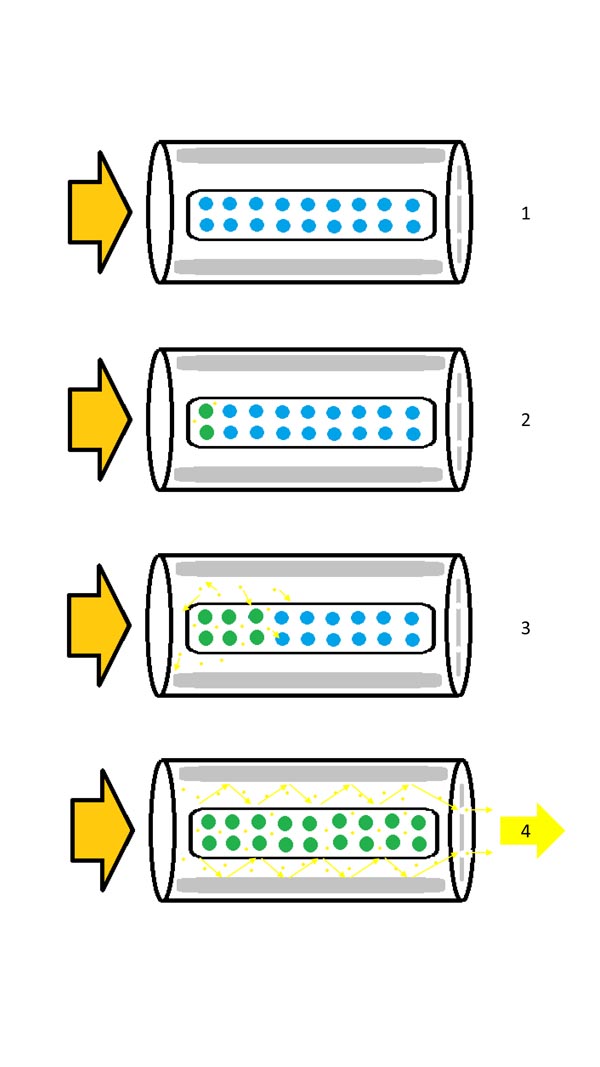
LASER; HISTORY OF ITS DISCOVERY AND APPLICATION
In 1900 Max Plank found, during his studies on the radiancy of light, frequency and temperature, that light is a form of electromagnetic radiation [1]. Previous to this the scientific knowledge of light was merely confined to observing its behaviour and characteristics. However, the mechanism by which light was generated was still unknown.
In 1917, Albert Einstein described the theory of stimulated emission in his publication “on quantum theory of radiation” [2]. This proposed that:
- Light travels in units of energy called photons, and;
- Most atoms exist in a standard ground energy stage (E0), however, a small number of these naturally occur at higher energy levels (E1, E2, E3, … En).
Einstein proposed that by adding energy to atoms in E 0 state, they could be excited to a higher energy level. Following their excitation to a higher energy level, they return or fall to their original energy level E0. During this process, the atoms emit the energy they acquired spontaneously in the form of photons or electromagnetic waves. This formed the basis of stimulated emission which is a core concept in the development of lasers (Fig. 2).
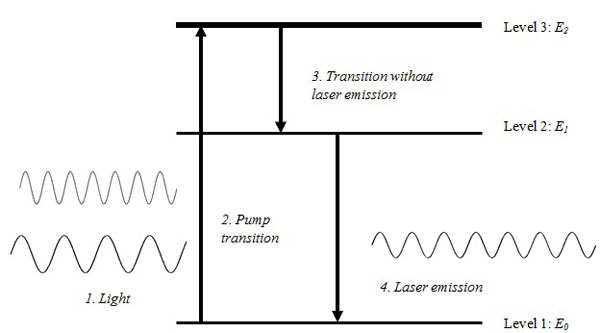
During the Second World War, scientists developed an interest in radar equipment and the microwave spectrum of light. In the post war era physicists were looking to amplify stimulated emission at a wavelength shorter than microwave spectrum, with Charles Townes building the first MASER in 1954 at the Columbia University in New York. At the same time in Lebedev laboratories in Moscow, Alexander Prokhorov and Nikolai Basov built a maser prototype. This led to the 3 men sharing the 1964 Nobel Prize for Physics for their research in the field of stimulated emission [3].
Forty three years after Einstein proposed his quantum theory of radiation, on 26th of May 1960, Dr. Theodor Harold Maiman demonstrated the first operational laser [4]. He introduced the first visible light laser using a silver coated ruby crystal, flashlamps and mirrors.
Gases such as nitrogen, Carbon dioxide (CO2) and combined Helium-Neon (He-Ne) were the key media used in the first generation of lasers. In 1961 the first He-Ne laser (wavelength 1.15 nm) was developed by Ali Javan [5]. In the same year the first Neodymium laser was invented [6]. Geusis et al. [7] published on the 1st laser using Yttrium-Aluminium-Garnet (YAG laser) in 1964. Other gas lasers were introduced by Patel (CO2 laser) [8] and Bridges (argon laser) [9]. It was not until 1966 where Soarokin and Lankard [10] built the first ruby-based dye laser, that liquid materials were used as the active medium.
As the spectrum of media has increased, so has the use of lasers across industry. It did not take long before lasers were incorporated into medicine for their therapeutic use. Today they are used across a breadth of medicine and surgical specialties, and their use and demand is only expanding.
LASER; LASER USE IN MEDICINE
The ancient Greeks and Egyptians are believed to have used sunlight as a therapy for treating illness. Today, lasers are applied in medicine for both diagnostic and therapeutic interventions, with their wavelengths ranging from infrared to UV [11]. A selected list of laser applications can be found in Table 1 [12-45].
Several factors mediate laser’s interaction with the human tissue: absorption, scattering, pulsed versus continuous wave. Water is the primary absorbing medium at wavelengths greater than 1 mm. Other absorbers include haemoglobin and proteins in the blood and melanin skin pigment. Similar to the way in which the absorption of different wavelengths of light changes the colour seen, the use of dyes allow a therapeutic manipulation and selective control of which tissues are targeted. An example of this would be in the treatment of oesophageal or urinary tract carcinomatous tumours where hematoporphyrin dye is used as a photosensitizer in photodynamic therapy to absorb in waves in the range of 630nm [46-49].
Speciality | Laser type examples | Use |
---|---|---|
Surgery in General | CO2, Nd:YAG, Ho:YAG | Haemostasis Sealing of Lymphatics Cutting |
Dermatology and Plastic Surgery | CO2, Nd:YAG, KTP, Diode, Pulsed dye, Ruby, Alexandrite, Erbium:YAG, Thulium fiber | Vascular/pigmented lesions [12] Hair removal Photo-rejuvenation Tattoo removal Body contouring/liposuction [13, 14] Scar therapy [15, 16] Lasar ablation/resurfacing e.g. acne/rhinophyma [17] Burn excision[18] |
ENT | CO2, Nd:YAG, KTP, Diode | Microlaryngeal/vocal chords Respiratory papillomas Middle ear Turbinates Polyps Sinus surgery |
Gastroenterology | Nd:YAG, Diode | Recanalisation of oesophagus Tumour excision/biopsy Actively bleeding ulcers Liver disease Stones; biliary [19] |
Gynaecology | CO2, Nd:YAG, Diode | External – condylomas Laparoscopic treatment Hysteroscopic treatment Foetoscopic treatment Colposcopic treatment Papillomas |
Neurosurgery | Infared | Peripheral neuropathy[20] Nerve repair [21] Tissue/tumour ablation [22] Discectomy [23] Cerebrovascular therapy [24,25] |
Opthamology | Nd:YAG, Argon, Krypton, Excimer, Erbium:YAG | Diabetic retinopathy Macular degeneration Retinal vein occlusion Iridectomy Corneal ablation Keratoplasty |
Oral and Maxillofacial Surgery | CO2, Nd:YAG, Diode | Tumour excision/biopsy Papillomas Peri-implantitis [26] |
Orthopaedic Surgery | Nd:YAG, Ho:YAG, Blue violet | Smoothing cartilage Knee surgery Lumbar disc decompression |
Thoracic Surgery | CO2, Nd:YAG, Excimer | Recanalisation trachea/upper airways Cardiac ablation Pacemaker implantation/Lead extraction [27] |
Urology | Nd:YAG, Ho:YAG, KTP, Diode, Pulsed dye | Stones; bladder/kidney Tumour excision/biopsy Ureteric strictures Bladder neck incisions Prostate coagulation [28] Prostate resection[28] |
Vascular | Nd:YAG | Endovascular ablation [29] Laser Doppler flowmetry |
Oncology | CO2, Nd:YAG, KTP, Gold vapour | Tumour excision/biopsy Photodynamic therapy (PDT) Palliative tumour ablation |
Pain Therapy | Diode, Low level laser therapy | Wound healing [30] Acute/Chronic pain therapy [31, 32] Acupuncture [33, 34] |
Microscopy/Genetics | Laser capture microdissection [35-37] | |
Other | Low level laser therapy | Psychology adjunct [38] Imaging [39] Implant fabrication [40-42] Onychomycosis [43] Musculoskeletal therapy [44] |
Scattering, on the other hand, limits the tissue penetration depth. Scattering describes when photons spread to outside the central target area/main beam. This could be desirable if treating a large tumour where a deep depth and large width are needed, but also harmful if treating a small, well defined area as normal cells can be damaged. The consequence of this is unpredictable and on a spectrum. This may have zero impact on the cell, or cause cell mutation, or death. This property is currently being studied extensively with the purpose of obtaining high resolution imagery in the screening for breast cancer [50].
The power range of lasers can be described as pulsed or continuous waveforms. Although some lasers are limited to one of these forms, the latter can be used to deliver a high energy quickly. This is useful for instance, in tissue ablation where evaporation of the top layer of cells is more useful than a continuous wave where the heat may be absorbed away from the target tissue. By adjusting the pulse frequency and thermal diffusion that occurs, the depth of laser effect can be determined.
The first continuous wave laser was introduced in 1970 in the form of an argon-pumped dye laser. There was huge excitement around creating more advanced lasers and extending their application. When researchers noted the ability of laser to burn through tissue it did not take long until they were applied in the surgical field. The laser energy was measured in “Gillette-units” referring to the amount of razors penetrated by one laser pulse [51]. Despite its destructive potential it was first used in medicine to do the exact opposite. Initially it was utilised by an ophthalmologist in a retinal repair [52, 53].
For the beginner in laser use, the treatment outcome of each laser type can be altered by modifying three aspects of the laser setup. It should be noted that some lasers have one of these settings set and so may be unable to be modified. The first feature that can be changed is the actual power generated by the laser and this is usually described in joules. This energy output can then be delivered to the target area in a short or a longer time period. This is what is described by the pulse duration. Lastly, some lasers such as the pulsed dye, the hand-piece can be changed. The hand-piece generally relates to the spot size or zone, which is to be treated. For the pulsed dye laser, which delivers the energy in a conical fashion, a wider spot size corresponds to a deeper ‘destruction pattern.’
The joules delivered and pulse duration can modify the tissue effect. A low density thermal energy can be used to induce tissue coagulation, which is useful for tissue debulking. Alternatively a higher density thermal energy given quickly causes vaporisation of the tissue cells, as well as a degree of coagulation necrosis to the surrounding cells. Modifying this delivery can result in an incisional laser function to a more destructive ablation.
LASER; SPECIFIC USES
Not long after their first introduction lasers conquered a place in the heart of medicine. Their applications became a significant part of diagnosis and treatment with the initial applications of ruby, argon, YAG, CO2, lasers taking place in 1964. Despite this, their potential dangers were noted early on some of which included CO2 laser tissue vaporization and YAG-induced coagulative necrosis. Visible lasers such as ruby and argon were noted to produce haemostatic coagulation.
These initial concerns were later to be utilised in different fields for a therapeutic effect. Today laser is used across many medical and surgical specialties, with varying media used in each practice.
Dermatology, specifically, has found a wide range of use for lasers. Examples include skin treatments such as spider naevi removal, hair removal, removal of tattoos and treating skin lesions [54-57]. Another well-known application is in urology where laser lithotripsy targets the removal of kidney and gallbladder stones.
Ophthalmology, general surgery and dentistry have also fully embraced laser therapy. Ophthalmologists were among the very first adaptors of this technology. This is understandable as the eye is designed to react to light! The fact that the eye is designed to allow light enter it allows many treatments of intra-ocular conditions. Argon lasers have been in use for numerous years in the treatment of retinal detachment and retinal haemorrhages, although the increasing availability of YAG and CO2 lasers, which can both incise tissue and coagulate bleeding, has led to their widespread and early use in general surgery [58-61]. In dentistry a different version of the YAG laser has been introduced for the purpose of reducing the post-procedural pain (Erbium[Er:YAG] as opposed to Neodymium [Ng:YAG]).
With the growing interest in lasers and advances in their development many diagnostic procedures such as flow cytometry with clinical applications such as immunophenotyping and measuring DNA content have developed throughout the years. Flow cytometry uses two laser beams to excite molecular particles in cells which can be utilised in cell analysis and sorting. Furthermore Flow cytometry is used in genetic engineering as it is capable of separating large numbers of chromosomes, which in turn can be used in creating recombinant DNA reference libraries.
The applications of lasers in medical imaging have been of great significance. New laser imaging technologies such as the optical coherence tomography (OCT) are being used in creating a special resolutions of tissue in the sub-millimetre range [62]. The newer version of OCT are specially accurate and sensitive that they are capable of detecting abnormalities such as atherosclerosis in its early stages. Another clinical application of OCT is its role in diagnosing a vast array of retinal macular pathologies. It has been shown to be able to produce accurate images of embryos, GI tract, skin and the vascular system. The resolution of MRI and ultrasound images are limited to 1mm range, although a new technique using spin polarised gases is being investigated to improve MRI images of the brain and lungs [63].
For those using lasers, there are complications and adverse effects described. Chiefly, for skin use in particular, these include erythema, blistering and burns to the skin and subsequent scarring. There are risks of thinning or thickening of the skin, infection and hypersensitivity to the treatment. Hypo and hyperpigmentation of the skin has been described, particularly if sun exposure occurs peri-therapy. Patients should also be made aware of partial- or non- resolution of symptoms and currently keloids. Scleroderma, systemic lupus erythema and isotretinoin use should be considered as contraindications to laser resurfacing.
LASER; FUTURE APPLICATIONS
One field of medicine which will laser would hopefully transform most, in both diagnosis and morbidity, is oncology. Raman spectroscopy is a relatively new use for laser technology and has shown promising results in imaging malignancy by differentiating the light reflected back by the normal and abnormal tissue in the human body. It provides a non-invasive imaging tool [64-66].
The use of current therapies will hopefully develop. Since its first use in dermatology and plastic surgery, its use has developed from benign skin lesions, through pigmented lesions, and is now being used in the treatment of primary and metastatic diseases, including squamous cell carcinoma and malignant melanoma [12, 54-57].
Photodynamic therapy is an application which may offer future treatment to not only skin malignancies but also the gastro-oesophageal, genito-urinary and respiratory tract neoplasias [47-50], with clinically based and laboratory experiments focusing on malignancy specific laser therapy [67-69]. Likewise, the use of photo-coagulation to treat breast and hepatic cancerous and benign lesions, potentially a tissue salvaging day case procedure, is being explored [47, 69].
The use of lasers in localised infections, involving bacteria and virus infected cell destruction, is theoretically sound and an area for future examination [47, 70-74].
In theory lasers can have a therapeutic effect against localised infections if light and photosensitising material can be delivered to the infected area. This makes treatment of infections such as MRSA-infected skin ulcers, or mouth ulcers with laser a very realistic possibility in the near future. Another possibility is the treatment of Helicobacter Pylori infection. It can be photosensitized with the use of methylene blue and photodynamic therapy can be applied endoscopically. Although the treatment of viruses can be technically more challenging, there is scope for sensitizing or even eradicating genital warts [47].
Tissue regeneration (skin, bone and cartilage) and fusion [75-80] is being commented on with signs that low-level laser therapy can induce and accelerate bone healing properties in particular [81-85]. Renno et al. [82] investigated the effect of laser irradiation on osteoblasts and malignant osteosarcomas in vitro and suggest that different cell lines respond differently to particular wavelengths and energy intensities. They demonstrated a significant increase in the proliferation of osteoblasts at 830-nm laser irradiation, but a decrease at 780-nm laser irradiation. Hawkins and Abrahamse [83] have suggested that with the correct number of exposures and energy density of helium-neon laser to wounded fibroblasts mitochondrial activity can be enhanced which can in turn positively influence cellular migration and proliferation without causing additional stress to already damaged or impaired cells.
The creation of the femtosecond laser [84-88] has helped reduce the amount of light exposure received by treated cells and tissues, and opens an option of generic therapy with new lasers being designed to create more accurate dissection. Hess et al. compared the cutting effects of scalpel, CO2 laser and the picosecond infrared laser-(PIRL) in cadaveric human vocal cords and concluded that the PIRL laser offers promising future clinical applications as it proved to cause close to no damage on the tissue that was being cut, avoiding coagulation and other forms of tissue insult [89].
Microneedles, created using lasers, as well as laser treatments to skin allow a more pain free administration of intravenous and subcutaneous medications compared to traditional methods, enhancing drug delivery [90-93].
This is specifically useful in the delivery of vaccines where a cutaneous route often induces a stronger immune response compared to a muscular route. Chen et al. discuss the advantage of using lasers in the delivery of vaccines, which can increase the immune response without any significant local or systemic adverse effects [90]. They argue that the desirable characteristics of such lasers, such as convenience, safety and their cost-effectiveness makes them a desirable subject for clinical studies in the near future.
The option for robotic assisted laser use is also an area gaining support [94, 95].
Another area is of course the laboratory use of lasers. The potential for lasers to hold and move microscopic units allowing individual cell isolation, and the ability of laser imaging allowing individual molecules to be highlighted and potentially targeted for pharmaceutical therapy will only develop in the future. In vivo microscopy is a possibility, again allowing less tissue destruction and increasing the specificity of surgical procedures [96].
The future offers a wider use of lasers, likely to involve less invasive and more specific targeting, potentially to a cellular level.
There has recently been a development in Intense Pulse Light Sources (IPL’s), which have similar indications to lasers but use a non-coherent ‘broad spectrum’ light ranging from the ultraviolet to infared regions. They are commonly used for hair removal currently but may have an increased spectrum of use [95, 96].
CONCLUSION
Lasers have developed greatly in their variability and application since Einstein’s theoretical ideas and the first use of a laser by Theodor Maiman at the end of the 1950’s [2, 4].
In terms of future innovation, laser use has the potential to be developed in regard to new wavelength bands, improvement in the average output power, peak pulse energy and power levels, as well as improvements in cost, power, and size efficiency.
These parameters will help medicine use lasers across a wider breadth of specialities with simpler, cheaper and smaller laser applications allowing a wider acceptance of laser use and introduction to smaller hospital units.
CONFLICT OF INTEREST
The author confirms that this article content has no conflict of interest.
ACKNOWLEDGEMENTS
Declared none.